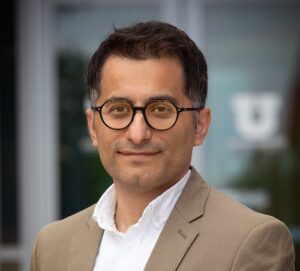
To put the physical scales into perspective, imagine a pebble sitting at the foot of Mt. Timpanogos, and then a single silicon atom on the surface of that pebble. The difference between a 12,000 ft. mountain and a rock the size of a pencil eraser is stark, but it’s about 3,000 times smaller than the one between the pebble and the atom. Even though these differences seem insurmountable, the laws of physics ensure that what happens on the nanoscale reverberates up to the length scales we encounter in our everyday experience. Those physical laws apply differently at different scales, however, complicating our ability to predict or control those impacts.
Salah Faroughi joined the U to bridge those gaps and address a key problem that he calls the “Energy-Environment dual challenge” along the way.
“The Energy-Environment dual challenge requires us to develop affordable energy sources while ensuring we do not compromise environmental integrity for future generations. It simply demands that we fuel our progress without burning our future. Bridging physics across scales unlocks a vast potential to design smart, multifunctional materials, enabling energy solutions that are both efficient and sustainable,” Faroughi says.
Faroughi recently joined Price’s Department of Chemical Engineering, though the nature of this work required a circuitous route through multiple other disciplines: mechanical engineering, geophysics, and civil and environmental engineering.
The core of Faroughi’s research approach is multiscale modeling techniques that can take those vanishingly small effects into account when simulating globe-spanning phenomena, such as climate change.
“Multiscale modeling requires enormous computing power, and the ocean of data it produces increasingly requires cutting-edge artificial intelligence techniques to explore and make sense of it all”, Faroughi says.
Faroughi is particularly interested in how cross-scale modeling can increase our understanding of “complex fluids,” or those imbued with microstructures like particles or polymers. Such fluids are promising because external magnetic or electrical fields can direct these microstructures to assemble into more complex, meso-scale structures. Those mesostructures, in turn, produce macroscopic functionalities, such as changes to the fluid’s viscosity, thermal properties, or other traits.
In addition to the differences in length scale, the time scales with which these mesoscopic structures form can range from seconds to milliseconds, all depending on the applied fields’ intensity and the atomic-scale properties of their constituent microstructures. If the dynamics occurring between these scales can be better understood, it could open the door to a variety of applications — surface skimming, targeted drug delivery and chemical delivery, soft robotics, additive manufacturing, energy materials and more.
Complex fluids are also ubiquitous in geological processes, both natural and engineered. One critical application is in hydraulic fracturing, which uses such fluids to open cracks and keep them from closure boosting hydrocarbon production. These fluids can also be engineered as working fluids in enhanced geothermal systems to boost heat mining.
“Another area that can increase our ability to shape the future of zero-emissions energy systems is cross-scale modeling of reactive multiphase flow in porous media.” Faroughi says.
Faroughi’s team is particularly interested in underground gas storage. Examples are injecting greenhouse gasses back into the fractured rock to sequester them from the atmosphere, or storing hydrogen in large quantity aimed at mitigating renewable energy curtailment. The latter has another dimension that adds more complexity than just cross-scale modeling, as microbial life that subsists on those chemicals must be accounted for as well.
“It is interesting to know that we can more-or-less model these phenomena on their respective scales, but when we zoom out or zoom in, we don’t yet have a solid way to connects the interactions across scales,” says Faroughi.
Faroughi plans to continue building up the robustness of these physics-based models; by weaving together disparate datasets, including ones that have been previously ignored, he is gradually increasing the resolution on these powerful predictive tools.
“We are actively developing cutting-edge computational methods and artificial intelligence techniques to advance cross-scale modeling and its versatile applications, which are crucial in shaping the future of zero-emissions energy systems”, Faroughi says.